For 80
years, physicists have been tricked by nature’s most subtle treat.
Ubiquitous but nearly undetectable, neutrinos are the ghostliest
fundamental particles.
In the
bewitching hours, as you seek safety in your bed, 500 trillion neutrinos
slice through your body every second. Produced by the Sun, they pass
through the Earth as if it were tissue paper, striking us from below,
and continuing upward into the heavens. And when you think the rising
Sun has vanquished vampires and apparitions, neutrinos strikes you from
above. Of this vast horde, less than one neutrino per year will actually
“hit” you; the rest pass through without even noticing you’re there.
Neutrinos are so ethereal that 90% can pass through a block of lead one light-year thick without the slightest interaction.
Yet neutrinos play an essential role in some supernovae, in powering stars, and in the creation of the atoms of which all living things are composed.
With the
discovery of the Higgs boson, physicists are now casting about for the
next Big Thing. For many, it’s time to search for ghosts, or at least
for neutrinos.
Neutrinos
have spooked physicists since Wolfgang Pauli first postulated them in
1930. Physicists had found that energy and momentum seemed to be lost in
the decays of certain radioactive elements. In the equivalent of a
quarterback’s Hail Mary pass, Pauli proposed that some
unobserved particles were carrying away the missing energy and momentum.
Fermi later named them “neutrinos” — Italian for “little neutral one.”
(No true Italian is that demure.)
Neutrinos
are the only particles of matter with no electric charge; hence they
don’t ionize material along their paths, so they escape direct
detection. Like ghosts, we can only infer their existence from the mess
left behind in their rare interactions with other matter, as seen below.
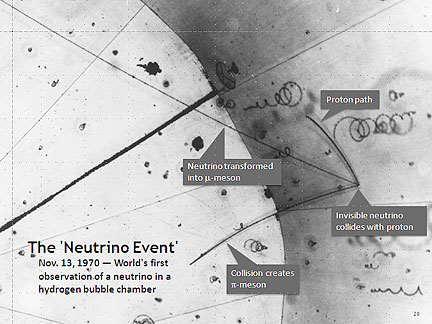
Muon neutrino entered a hydrogen bubble chamber from right, struck a proton, and produced a muon and pion.
Neutrinos
are the only fundamental particles (those not made of anything
“smaller”) whose masses remain unknown. We think they are a million
times lighter than any other particle of matter. Why so different? It’s
another mystery.
In 1956, 26 years after Pauli’s Hail Mary, the first neutrino was actually detected by Clyde Cowan and Fredrick Reines, for which they won the 1995 Nobel Prize.
As if one
neutrino wasn’t spooky enough, Leon Lederman, Jack Steinberger, and
Melvin Schwartz (my thesis advisor) discovered a second type in 1962,
for which they won the 1988 Nobel Prize.
Another mystery is why the Nobel committee rewarded the discoverers of the second neutrino type seven years before rewarding the discoverers of the first type.
Yet a third
neutrino type was discovered by the DONUT collaboration at the U.S.
Fermi National Lab in 2000. The name DONUT seems odd. It stands for
Direct Observation of NU Tau, but doesn’t DONUT sound like they’re
missing something? No Nobel for DONUT, at least not yet.
All of that
adds up to three types of ghostly neutrinos, named for their associated
charged cousins: the electron, muon, and tau, in the order of their
discovery.
While all
other fundamental particles are content with one identity, neutrinos
schizophrenically morph from one type to another and back again. Such
“flavor oscillations” would be impossible if all neutrino types had zero
masses, as physicists long believed.
Particle
physicists haven’t successfully measured the mass of the neutrino. But
strangely, cosmologists have. By measuring the total energy distribution
of the universe, cosmologists have shown that the total mass of one
neutrino of each of the three types is 2.5 million times less than the
mass of one electron, the next lightest particle. Amazingly, the best
way to measure the mass of the lightest particles is to measure the mass
of universe and subtract everything else (well, sort of).
Immense
numbers of neutrinos are produced when massive stars explode in
spectacular supernovae and form neutron stars. In 1987, three neutrino
detectors (in Japan, in a Morton Salt mine near Lake Erie, and in
Russia’s Caucasus Mountains) detected a total of 24 neutrinos during a
13-second burst. These neutrinos came from SN1987a, the nearest
supernovae of the last 400 years. This event created a new field of
science: neutrino astronomy.
Supernovae
can briefly shine brighter than billions of normal stars. Yet physicists
estimate that only 1% of the energy released by supernovae, like
SN1987a, is in the form of visible light; 99% is in the form of
invisible neutrinos. The neutrinos are produced during the initial
collapse, while the supernova’s visible light comes mostly later as
radioactive nuclei decay. The result is SN1987a’s neutrinos arrived
several hours before its light. Astronomers hope to use neutrino
detectors as early warning systems for supernovae.
Stars,
including our Sun, produce neutrinos in their cores as they fuse
hydrogen into helium. For each helium nucleus produced, two protons
transform into two neutrons, two antielectrons, and two electron
neutrinos. This nuclear fusion process releases a colossal amount of
energy that powers the stars and enables life on Earth. Additional
fusion reactions produce carbon and other atoms that life requires.
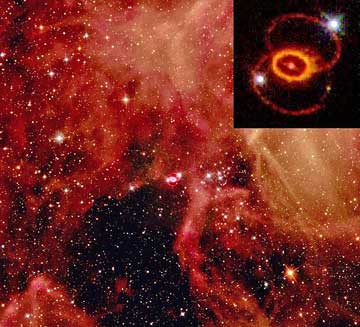
Remnant of Supernova SN1987a, first seen with neutrinos
Neutrinos are friendly ghosts.
More on neutrinos in another newsletter.....
Have a Safe & Happy Halloween
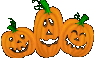
Best Regards,
Robert
October 31st, 2013
Note: Previous newsletters can be found on my website.
|