My December newsletter announced the first-ever detection of gravitational waves from the merger of two neutron stars. That event has since been meticulously analyzed in a research paper cited below.
It amazes me how much was discovered from one cataclysmic collision that was seen with very different instruments. Three ground-based observatories — both U.S. LIGO sites and VIRGO in Italy — detected the event’s gravity waves (GW) while two space telescopes — FGST (Fermi Gamma-ray Space Telescope) and INTEGRAL (INTErnational Gamma-Ray Astrophysics Lab) — observed a gamma ray burst (GRB) this event also produced.
The first step was establishing that everyone witnessed the same collision.
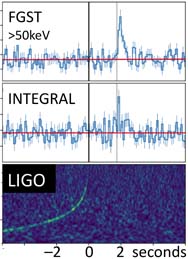
As shown here, the GRB arrived 1.74 seconds after the GW peak, which corresponds to the instant the neutron stars collided.
FGST has detected a GRB about once every 8 days. So the chance that a GRB accidentally occurred with ±1.74 seconds of the GW is 1 in 200,000.
)
FGST localized the origin of the GRB to about 1% of the entire sky (purple area in sky map at right). LIGO and VIRGO did far better, localizing the GW origin to 28-square-degrees (green area), or 0.07% of the entire sky. The chance of an accidentally alignment is 1 in 100.
The yellow star above (in the green area) is the event’s location seen in optical telescopes, which have much better resolution than either GW or GRB detectors.
The authors next compared the velocities of gamma rays and gravity waves. Using the observed 1.74-second arrival time difference, the estimated 140-million-light-year source distance, and assuming simultaneous emission, their velocity difference is no more than 1 in 2500 trillion.
If Einstein is correct, and gamma rays and gravity waves have exactly the same velocity, the speed of light, the GRB must have been emitted 1.74 seconds after the GW peak. Some short delay does seem reasonable; astrophysicists theorize gamma ray bursts arise from shock waves among particles ejected from a mammoth explosion — in this case, the collision of two neutron stars, each about 40% more massive than our Sun, and each moving at nearly half the speed of light.
With more such events at various distances from Earth, we will be able to separate the arrival time differences due to GRB emission delays and those due to wave velocity differences — the latter are proportional to distance, while the former are distance-independent.
This constraint on the GW-GRB velocity difference also sets limits on the possible violations of Einstein’s equivalence principle and Lorentz invariance, both foundations of his theories of relativity. The authors find that the former must be less than 1 in 1 million, while the later must be less than 1 in 25 trillion.
Finally, the authors compute rough estimates for joint GW-GRB detection rates: 0.1 to 1.4 per year with current technology, and to 0.3 to 1.7 per year when LIGO and VIRGO achieve full design performance. It may take a decade or more to collect a statistically significant sample.
As frequently occurs, this event raises as many questions as it answers — scientists never run out of questions.
We wonder what became of the colliding neutron stars? LIGO measured their pre-merger total mass to be 2.74 ± 0.04 solar masses, which is squarely in no-man’s-land. The collision remnant might be the least massive black hole, or the most massive neutron star, ever observed. If the merged object immediately formed a black hole, thereby becoming invisible, it probably would not emit a GRB. If the merged object remained a neutron star, why don’t we see any continuing gamma ray emission? Perhaps the merged object became a neutron star for a few seconds before collapsing to a black hole.
Also puzzling are the closeness and dimness of the GRB source. The source of this event is 100 times closer to Earth than similar GRBs, those of short duration (up to 2 seconds). Also, its brightness is up to 1 million times less than other short GRBs. There are numerous possible explanations that only more data can resolve.
One thing is certain — such discoveries are only possible because of multi-messenger astronomy, seeing the universe through several different lenses.
This research, published in DOI and Astrophysical Journal Letters, was performed by B. P. Abbott and nearly 800 others authors from 183 institutions.
)
Best Regards,
Robert
March 20th, 2018
Note: Previous newsletters can be found on my website
|